Light Penetration in Water
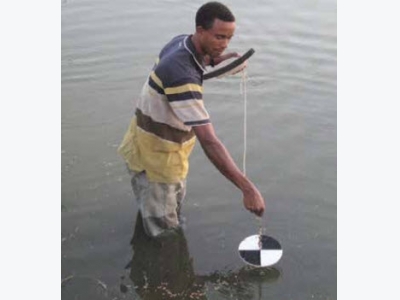
The depth of the photic zone, the layer of water receiving 1% or more of incident light, can be estimated with Secchi disk visibility.
Summary:
Light penetrating water is scat-tered and absorbed exponentially as it passes downward. The pres-ence of dissolved organic matter and suspended solids further impedes light penetration, and different types of solids absorb different wavelengths. Light pen-etration into water also is the en-ergy source for photosynthesis by phytoplankton and other aquatic plants. Photons of light absorbed by water warm the water, decreas-ing with depth. The varying den-sity of the water can create layers of thermal stratification within water bodies.
Sunlight consists of the entire spectrum of electromagnetic radiation, which includes gamma, X, ultraviolet, visible, infrared, micro- and radio waves. The major part of solar radiation is in the form of visible and infrared rays that vary in wavelength.
Sunlight that strikes the earth’s surface is either absorbed or reflected. The trans-parency of water surfaces varies. It is greater for clear water, when the water surface is smooth, and between mid-morning and mid-afternoon, when the sun’s rays are nearest to vertical with the earth’s surface. Latitude and season also affect the angle of the sun’s rays. When the angle of incidence of the sun’s rays is 60° or less, less than 10% of incident sunlight normally is reflected by a water surface.
The percentage of incident light reflected by a surface is called the albedo. The annual albedos of water bodies range from 5 to 7% at the Equator to 12 to 13% at 60° latitude. On a monthly basis, the albedo of water varies little with time of year at the Equator, but at 60° latitude, the albedo is around 5% at equinox in March and September, and around 55% at the beginning of winter.
Light Penetration
Light penetrating a water surface is scattered and absorbed or quenched as it passes downward. Water scatters but does not absorb ultraviolet light. It absorbs infrared light quickly – little infrared light penetrates more than 2 m. Light is quenched rather quickly even in clear water. Only about 25% of incident light reaches a depth of 10 m in the open ocean, where water is very clear.
The visible spectrum consists of wavelengths between 390 and 750 nano-meters (nm, Table 1). One nanometer is equivalent to 1 billionth of a meter. The rate of visual light attenuation in water is greatest for red and orange rays, less for violet rays and least for yellow, green and blue rays. The presence of dissolved organic matter and suspended solids fur-ther impedes light penetration, and dif-ferent types of solids preferentially absorb at different wavelengths.
Phytoplankton absorb light best within the red and orange part of the spectrum, but they absorb other colors to a lesser degree. Dissolved organic matter absorbs blue, violet and ultraviolet light most strongly. Suspended mineral matter tends to absorb light uniformly across the visible spectrum, while dissolved inor-ganic substances do not interfere with light absorption by water. Salinity, there fore, does not have a significant impact on underwater light.
Table 1. Categories of light rays and colors in the electromagnetic spectrum of sunlight.
Entire Spectrum | Visible Spectrum | ||
Ray Type | Wavelength | Ray Color | Wavelength |
Gamma | Less than 0.01 nm | - | - |
X | 0.01-10.00 nm | Violet | 390-450 nm |
Ultraviolet | 10.00-389.00 nm | Blue | 450-495 nm |
Visible | 390.00-750.00 nm | Green | 495-570 nm |
Infrared | 759.00-106 nm | Yellow | 570-590 nm |
Micro | 106 -109 nm | Orange | 590-620 nm |
Stratification
When photons of light are absorbed by water, they warm the water. The amount of heat imparted to the water decreases with increasing depth. Of course, water re-radiates long-wave radia-tion to the atmosphere, and an equilib-rium between incoming and outgoing radiation tends to be achieved, placing limits on water temperature changes over a 24-hour or longer period.
In many water bodies, heat is gained in the upper layer faster than it can be mixed into deeper water by wind-driven water cur-rents. This results in an upper layer of warm water of lower density overlaying a deeper layer of cooler water of greater density. If the difference in density between the two layers becomes so great that surface water cannot be mixed with deeper water by wind action, thermal stratification occurs. In a thermally stratified water body, the upper layer is called the epilimnion, the lower layer is referred to as the hypolimnion, and the layer across which temperature changes rapidly is known as the thermocline.
When the surface layer cools and increases in density, or wind and rain result in stronger mixing, thermal stratifi-cation disappears. Depending on the characteristics of water bodies and cli-mate and weather conditions, thermal stratification can develop and collapse on a daily, seasonal or sporadic schedule. Some lakes in tropical climates stratify for very long periods.
Aquaculture ponds usually are shallow, and stratification that develops on warm, calm days does not persist at night, when heat is lost to the overlaying air. Of course, in aerated ponds, aerator-generated water currents keep waters thoroughly mixed.
The hypolimnion of a thermally strat-ified water body often is devoid of dis-solved oxygen. Thermal destratification – especially if it is sudden – can cause dissolved oxygen depletion and lead to fish kills. Sudden destratification in lakes containing fish cages is a significant risk.
Photosynthesis
Light penetration into water also is the energy source for photosynthesis by phytoplankton and other aquatic plants. Plants use red and orange light best, but they also use other parts of the visible spectrum. Wavelengths between 400 and 700 nm are called photosynthetically active radiation (PAR), and underwater light meters capable of measuring PAR are available.
Because photosynthesis is a chemical reaction mediated by energy from pho-tons of light captured by chlorophyll and other light-sensitive pigments in plant cells, photons are treated as if they are molecules in measuring PAR.
Photic Zone, Secchi Disk
As a general rule, phytoplankton and other aquatic plants cannot survive at light intensities below 1% of light received at the surface – whether mea-sured as total light or PAR. The layer of water receiving 1% or more of incident light is known as the photic or euphotic zone. Because light is quenched exponen-tially with depth, the percentage of inci-dent PAR decreases rapidly (Figure 1). Incidentally, the depth profile for attenu-ation of total light would be almost iden-tical to that for PAR.
The underwater light profile illus-trated in Figure 1 is for noon on a clear day in a tropical aquaculture pond with a phytoplankton bloom. Light penetration to different depths was estimated with the Lambert’s law equation. Less than 20% of incident light reaches 0.50 m, a little over 2% reaches 1.00 m, and the photic zone is only 1.35 m thick – a fairly typical situation in an aquaculture pond.
In ponds with aeration, induced water circulation continually brings phytoplank-ton from deeper water into the photic zone and vice versa. This phenomenon has basically the same effect as increasing the thickness of the photic zone – it increases the amount of photosynthesis per unit surface area in a water body.
In lakes, the depth of the photic zone typically corresponds to the depth at which thermal stratification occurs. There is no photosynthesis in the hypolimnion to provide dissolved oxygen. Lakes in which hypolimnetic dissolved oxygen depletion occurs are classified as eutrophic – mean-ing rich in nutrients – as opposed to oligo-trophic lakes that are nutrient-poor.
The depth of the photic zone can be estimated with Secchi disk visibility. The value 1.7 divided by the Secchi disk visi-bility in meters has been reported by sev-eral researchers to provide a good estimate of the light extinction coefficient (K) for use in the Lambert’s law equation. Using this method for obtaining K and solving the Lambert’s law equation for depth of 1% light penetration suggests that the thickness of the photic zone is about 2.7 times the Secchi disk visibility. The depth of the photic zone has been variously reported as two to three times the Secchi disk visibility based on studies of plant growth, but the value of 2.7 is a good one for general purposes.
Turbidity created by phytoplankton growth in ponds often is a means to avoid growth of underwater macrophytes. At a typical Secchi disk visibility of 40 to 50 cm in aquaculture ponds, a minimum depth of 110 cm is necessary to avoid enough illumination at the bottom for plant growth.
Related news

Productivity increases in aquaculture ponds in re-sponse to nutrient inputs of fertilizer and feed. These in-puts, however, increase the demand for oxygen

Mechanical water circulation can prevent stratifica-tion and provide more dissolved oxygen near pond bottoms

Aquaculture ponds should not be deeper than about 2.0 m to minimize the probability of thermal stratification.